5901 Botham Jean Blvd, Dallas, TX 75215
How Does a Magnet Work: Understanding the Basics
May 9, 2025Magnets are a part of our everyday life, from refrigerator doors to advanced medical equipment. These remarkable objects generate invisible forces that can attract certain metals from a distance without physical contact. This fundamental property has fascinated scientists and curious minds for centuries.
At its core, a magnet produces a magnetic field—an invisible region of influence extending around it. This field contains lines of force that always travel in a specific pattern. These lines exit from the magnet’s north pole and enter through its south pole, creating a continuous loop. When you place iron filings around a magnet, they reveal this otherwise invisible pattern dramatically.
All magnets have two poles—north and south—which follow a simple rule: opposite poles attract while like poles repel. Place the north pole of one magnet near the south pole of another, and they’ll pull together with surprising force. Conversely, two north poles placed near each other will push apart. This behavior explains why breaking a magnet in half creates two smaller complete magnets rather than separate north and south pieces.
What Are Magnetic Domains and How Do They Work?
Magnetic domains are microscopic regions within ferromagnetic materials like iron, nickel, and cobalt. Each domain acts as a tiny independent magnet with its own north and south poles. These domains form when a material cools below a temperature known as the Curie temperature.
Within each domain, thousands to trillions of atomic magnetic moments align uniformly in one direction. This internal alignment creates a strong magnetic field within that specific region. Think of each domain as a small country where all the citizens agree to point in the same direction.
In an unmagnetized ferromagnetic material, these domains point in random directions, causing their magnetic fields to cancel each other out. This explains why a piece of iron doesn’t naturally stick to your refrigerator despite containing magnetic elements.
Domain Alignment and Magnetization
When an external magnetic field is applied to a ferromagnetic material, domains that align with the external field begin to grow, while those opposing it shrink as domain walls move through the material.
This process continues until most domains point in the same direction, resulting in a material with a strong net magnetization. The stronger the external field, the more complete this alignment becomes. At saturation, virtually all domains align with the applied field.
This phenomenon explains why breaking a bar magnet creates two smaller magnets, each retaining its own collection of aligned domains with north and south poles.
Why Domains Form
Magnetic domains exist because they represent the lowest energy state for ferromagnetic materials. A single-domain structure would create a large external magnetic field, requiring substantial magnetostatic energy.
By dividing into multiple domains with different orientations, the material reduces this energy. Magnetic field lines can loop between adjacent domains rather than extending far outside the material, minimizing the system’s total energy.
Domain sizes typically range between 10-4 and 10-6 meters, balancing the energy saved by reducing external fields with the energy required to create domain walls where magnetization changes direction.
Domain State | Description |
---|---|
Unmagnetized | Domains are oriented randomly, resulting in no net magnetic field. |
Magnetized | Domains align with an external magnetic field, creating a net magnetic field. |
Domain Walls | Boundaries between domains where magnetization changes direction. |
Domain Wall Movement | Occurs under external forces; can be pinned by defects, maintaining magnetization. |
Curie Temperature | The temperature above which a material loses its permanent magnetic properties. |
Domain Wall Movement
Domain walls—the boundaries between regions of different magnetization—are crucial in magnetic behavior. These walls can move when subjected to external forces, but when they encounter defects or impurities in the crystal lattice, they can become pinned in place.
This pinning effect explains how permanent magnets maintain their magnetization. Even when the external field is removed, domain walls remain locked in their aligned position, preserving the magnetic properties.
Heat, mechanical shock, or alternating magnetic fields can provide enough energy to free these pinned walls, allowing domains to return to a more random orientation, effectively demagnetizing the material.
The study of magnetic domains, called micromagnetics, has numerous applications in data storage technology, electrical transformers, and magnetic sensors. Understanding domain behavior helps engineers develop more efficient and powerful magnetic materials for recycling equipment and other industrial applications.
How Are Different Types of Magnets Created?
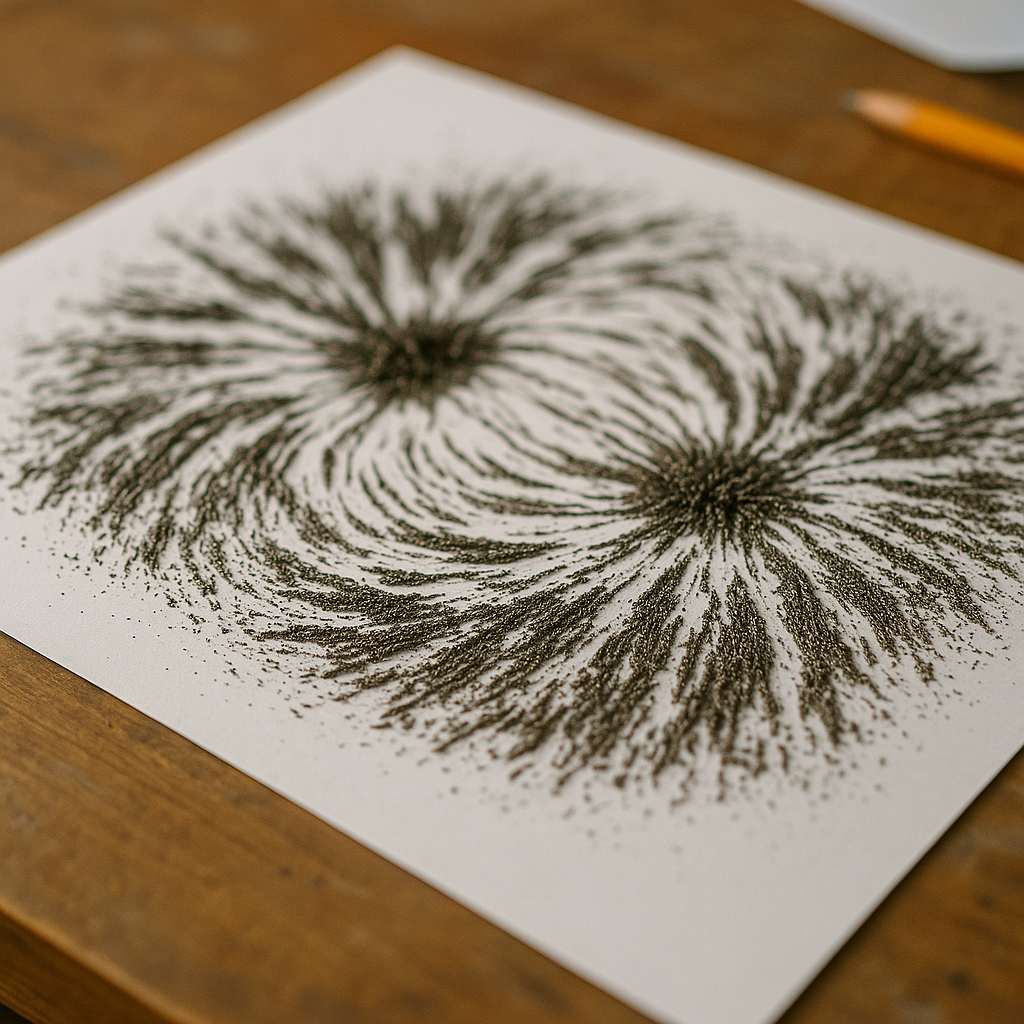
The creation of magnets has evolved from ancient discoveries to sophisticated modern manufacturing. Lodestone, a naturally occurring magnetite, was the first magnetic material discovered by humans over 5,000 years ago. Ancient civilizations used this natural magnet to magnetize iron by rubbing it against metal objects.
Today, manufacturers use several methods to create magnets with varying strengths and properties. The alignment of microscopic regions called magnetic domains is the key principle behind all magnetization techniques. In an unmagnetized material, these domains point in random directions. Creating a magnet involves aligning these domains in the same direction.
Common Magnetization Methods
The most widespread technique for creating magnets involves placing metal in a strong magnetic field. This field exerts torque on the material, encouraging the domains to align in a uniform direction. The process isn’t instantaneous. A slight delay, known as hysteresis, occurs between applying the field and the domains beginning to move.
Striking a ferromagnetic material with a hammer while it’s aligned in a north-south direction offers another approach. This physical jarring can cause domains to weakly align. Some metalworkers in earlier centuries used this technique to create simple magnetic tools.
Passing an electrical current through metal provides a third method for creating magnets. This principle forms the basis for electromagnets, where the magnetic field exists only while current flows through the material.
Magnet Strength and Permanence
A magnet’s strength directly relates to the force used to move the domains during creation. Stronger fields produce more powerful magnets. Scientists measure this strength in units like tesla or gauss.
The permanence of a magnet depends on how difficult it was to align the domains initially. Materials that resist magnetization tend to retain their magnetic properties longer after creation. This quality, known as retentivity, varies significantly between different magnetic materials.
Heat significantly affects magnetic properties. Heating a magnet above its Curie point will cause it to lose magnetism as the thermal energy disrupts domain alignment. This principle allows for demagnetization when needed.
Types of Manufactured Magnets
Different materials produce magnets with varying characteristics. Ferrite or ceramic magnets contain iron oxide in a ceramic composite. These affordable magnets appear in refrigerator decorations and elementary school science experiments.
Alnico magnets, developed in the 1930s, combine aluminum, nickel, and cobalt. These magnets offer stronger fields than ceramic types but less strength than rare-earth magnets.
Neodymium magnets represent the strongest commercially available option. These powerful magnets contain iron, boron, and the rare-earth element neodymium. Their exceptional strength makes them essential in modern electronics, despite their higher cost.
Samarium cobalt magnets, developed in the 1960s, combine cobalt with the rare-earth element samarium. These magnets maintain their performance at higher temperatures, making them valuable for specialized applications.
Magnet Type | Composition | Strength | Applications |
---|---|---|---|
Neodymium (NdFeB) | Neodymium, Iron, Boron | Strongest | Electric motors, hard drives, headphones |
Ferrite (Ceramic) | Iron Oxide, Barium/Strontium Carbonate | Moderate | Speakers, magnetic toys |
Samarium-Cobalt (SmCo) | Samarium, Cobalt | High | Aerospace, military applications |
Alnico | Aluminum, Nickel, Cobalt | Moderate | Musical instruments, meters |
Understanding these manufacturing principles has enabled the creation of increasingly powerful magnets. These advancements support technologies from simple compasses to sophisticated medical equipment like MRI machines. The evolution of magnet manufacturing continues to expand possibilities in recycling operations, renewable energy systems, and countless other applications.
Why Do Magnets Attract Certain Materials?
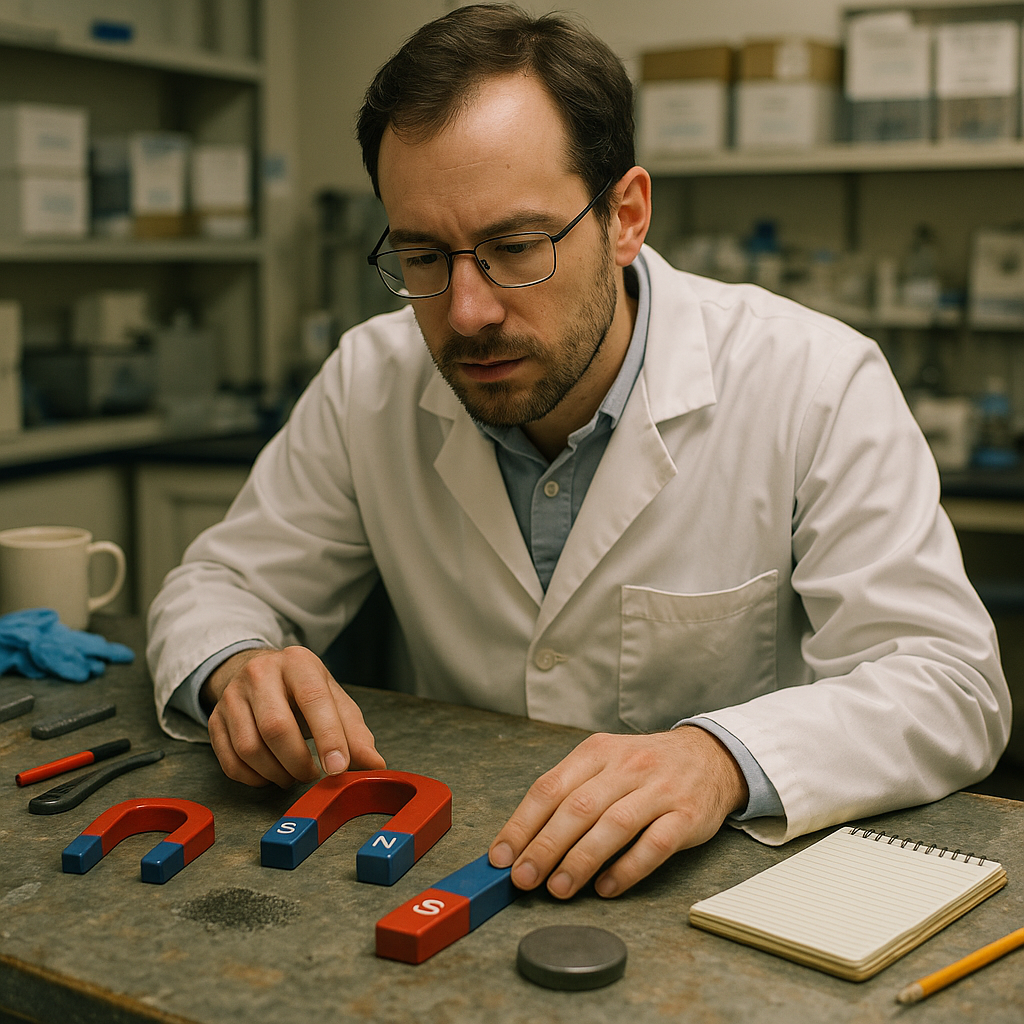
Magnets attract certain materials due to a fascinating atomic phenomenon. Materials like iron, nickel, and cobalt have unpaired electrons that create tiny magnetic fields at the atomic level. These unpaired electrons spin in the same direction, resulting in a cumulative magnetic effect.
Ferromagnetic materials are notable for their strong magnetic attraction. These materials have a unique atomic structure where electrons aren’t evenly paired. Iron has four unpaired electrons, cobalt has three, and nickel has two. Their unpaired electrons form microscopic magnetic domains that align when exposed to an external magnetic field.
When a magnet approaches iron, the magnetic domains within the iron align with the magnet’s field, creating an attractive force between the two objects. The more domains that align, the stronger the attraction becomes.
The Science of Electron Spin
At the atomic level, electrons behave like tiny magnets with their own north and south poles. In most materials, electrons pair up with opposing spins, canceling out their magnetic effects. It’s like placing two small magnets facing opposite directions – they neutralize each other.
Ferromagnetic materials like iron are different. They contain unpaired electrons that maintain their magnetic properties. These unpaired electrons create magnetic moments that can align with external magnetic fields.
This alignment principle explains why magnets stick to refrigerators but not to aluminum cans. Materials with unpaired electrons that can easily align with a magnetic field are attracted to magnets.
Different Types of Magnetic Responses
Not all materials respond to magnets in the same way. Materials fall into three main categories based on their magnetic behavior.
Ferromagnetic materials show strong attraction to magnets. Iron, nickel, and cobalt are classic examples. These materials can retain some magnetism even after the external magnetic field is removed. Many common objects like paperclips and steel tools contain ferromagnetic materials.
Paramagnetic materials display weak attraction to magnets. Materials like aluminum, platinum, and oxygen have unpaired electrons. However, these electrons don’t align as easily as those in ferromagnetic materials. The attraction is often too weak to notice without sensitive equipment.
Diamagnetic materials slightly repel magnets. Materials like copper, silver, and gold have no unpaired electrons. When exposed to a magnetic field, they generate a weak opposing field. This repulsion is usually very subtle. Water is diamagnetic, which is why extremely strong magnets can levitate water droplets in laboratory conditions.
Practical Applications of Magnetic Attraction
Understanding why certain materials are attracted to magnets has led to numerous practical applications. Recycling facilities use magnetic separation to efficiently sort ferrous metals from other waste materials. The same property allows scrapyards to separate valuable metals.
Magnetic resonance imaging (MRI) machines rely on the magnetic properties of hydrogen atoms in water molecules within our bodies to create detailed medical images. The technology depends entirely on how different materials respond to magnetic fields.
Electric motors and generators convert between electrical and mechanical energy using magnetic attraction principles. The interaction between electromagnets and ferromagnetic materials creates motion that powers everything from small toys to electric vehicles.
Credit cards, computer hard drives, and speakers all use magnetic properties to function. Data storage relies on creating tiny magnetized areas that represent digital information.
The recycling industry particularly benefits from magnetic properties. Separating ferrous metals from non-magnetic materials is a fundamental step in processing recyclable materials efficiently. Large electromagnets can be turned on to attract ferromagnetic materials and turned off to release them into separate collection areas.
The same qualities that make a material good for creating magnets also make it attracted to magnets. This fundamental physical property continues to drive technological innovation across numerous industries.
Conclusion: The Fascinating World of Magnets
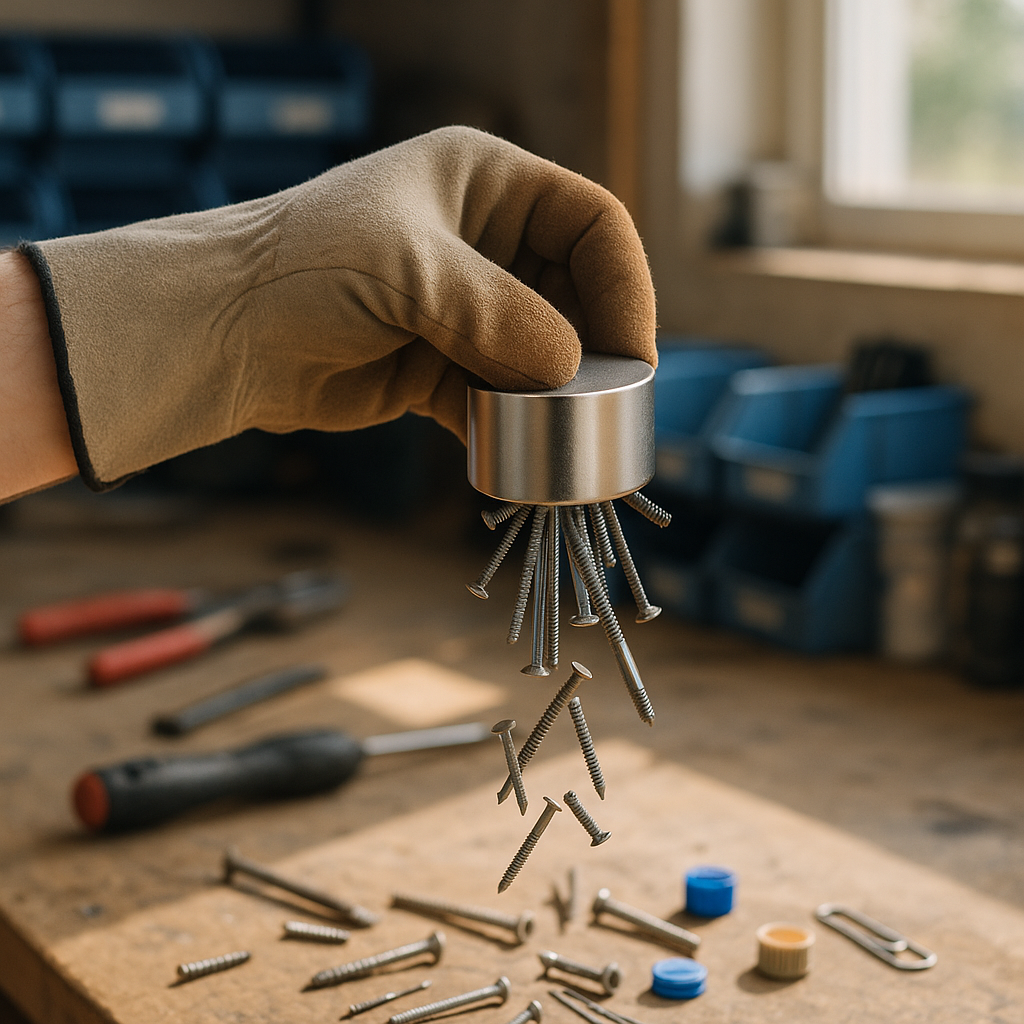
Magnets are remarkable components that power our modern technological landscape. These fundamental forces of nature are indispensable across diverse industries. From the electric motors in household appliances to sophisticated MRI machines in hospitals, magnetic technology shapes many aspects of our daily lives. The principles of magnetic domains and electron behavior allow these powerful tools to function through precise attraction and repulsion mechanisms.
As technological innovation advances, applications of magnetic properties continue to expand into exciting new territories. Magnetic levitation promises revolutionary transportation systems. Quantum computing harnesses magnetic principles for unprecedented computational power. Even sustainable energy solutions like wind turbines rely heavily on magnetic components. For any recycling or waste management needs related to electronic components containing magnets, contact Okon Recycling at 214-717-4083.